Bacteria: Our Ancestors and Coevolutionary Partners
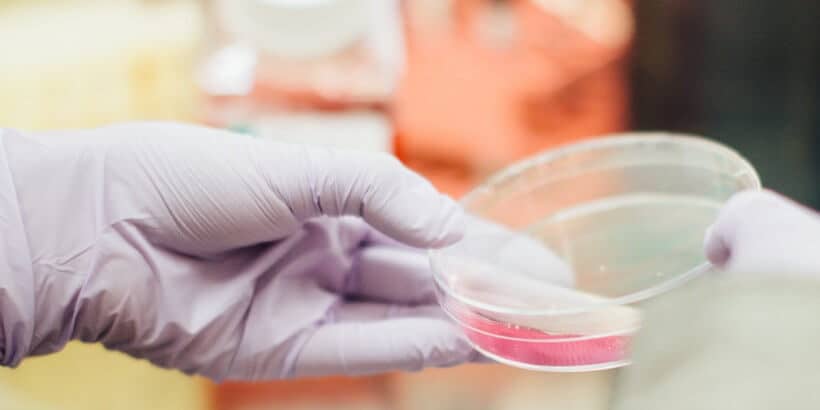
Fermentation is the transformation of food by various bacteria, fungi, and the enzymes they produce. People harness this transformative power in order to produce alcohol, to preserve food, and to make it more digestible, less toxic, and/or more delicious. It’s played an instrumental role in human cultural evolution and has become a cultural phenomenon of sorts. That said, it is important to recognize that fermentation is a natural phenomenon much broader than human culinary practices; cells in our bodies are capable of fermentation. In other words, humans did not invent or create fermentation; it would be more accurate to state that fermentation created us.
The following excerpt is from The Art of Fermentation by Sandor Ellix Katz. It has been adapted for the web.
Biologists use the term fermentation to describe anaerobic metabolism, the production of energy from nutrients without oxygen. Fermenting bacteria are thought to have emerged relatively early from the primordial prebiotic soup, before the atmosphere had a sufficient concentration of oxygen to support or evolve aerobic life-forms. “In the first two billion years of life on Earth, bacteria—the only inhabitants—continuously transformed the planet’s surface and atmosphere and invented all of life’s essential, miniaturized chemical systems,” writes biologist Lynn Margulis.3 The research of Margulis and others has convinced many biologists that symbiotic relationships between fermenting bacteria and other early single-cell life-forms became permanently embodied as the first eukaryotic cells that plants, animals, and fungi comprise.4 As Margulis and Dorion Sagan explain in their book Microcosmos, the symbiosis may have begun as a predator-prey relationship:
Eventually some of the prey evolved a tolerance for their aerobic predators, which then remained alive and well in the food-rich interior of the host. Two types of organisms used the products of each other’s metabolisms. As they reproduced inside the invaded cells without causing harm, the predators gave up their independent ways and moved in for good.5
Evolution derived from such symbiosis is known as symbiogenesis. Microbiologists Sorin Sonea and Léo G. Mathieu elaborate on the concept: “Symbiogenesis with thousands of different bacterial genes has decisively enriched the limited metabolic potential of eukaryotic organisms, accelerating and facilitating their adaptation much more than would have been achieved by random mutation alone.”6
Bacterial fermentation processes have been part of the context for all life.
Fermentation plays such a broad and vital role in nutrient cycling that all beings coevolved with it, ourselves included. Through symbiosis and coevolution, bacteria fused into new forms, spawning all other life. “For the past [bil- lion] years, members of the Bacteria superkingdom have functioned as a major selective force shaping eukaryotic evolution,” state molecular biologists Jian Xu and Jeffrey I. Gordon. “Coevolved symbiotic relationships between bacteria and multicellular organisms are a prominent feature of life on Earth.”7 The importance of bacteria and our bacterial interactions cannot be overstated. We could not exist or function without our bacterial partners.
Like all complex multicellular life-forms, the human body is host to an elaborate indigenous biota. Some geneticists argue that we are “ a composite of many species,” with a genetic landscape that encompasses not only the human genome but also those of our bacterial symbionts.8 In our bodies, bacteria outnumber the cells containing our unique DNA by more than 10 to 1.9 The vast majority of these bacteria—a mind-boggling 100 trillion (1014) in number—are found in our intestines.10 Bacteria break down nutrients we would not otherwise be able to digest11 and play an important role, just beginning to be recognized, in regulating the balance between energy use and storage.12 Intestinal bacteria produce certain necessary nutrients for us, including B and K vitamins.13 They provide us with vital defense by “outcompeting invading pathogens for ecological niches and metabolic substrates.”14 In addition, intestinal bacteria are able to modulate “expression” of some of our genes, related to “diverse and fundamental physiological functions,”15 including immune response. “Evidence of an active dialogue is rapidly unfolding” between intestinal bacteria and the immune cells of the intestine linings.16
That’s just the bacteria in our intestines. On our bodies’ surfaces, microbial communities exist in a great range of distinct niches. “For example, hairy, moist underarms lie a short distance from smooth dry forearms, but these two niches are likely as ecologically dissimilar as rainforests are to deserts,” observed a 2009 study of the genetic diversity of skin bacteria.17 Bacteria inhabit all our surfaces, particularly the warmer sweaty places that stay moist, as well as our eyes, upper respiratory tract, and orifices; more than 700 species have been detected in the healthy oral cavity.18
Even our reproduction requires fermentation. The human vagina has been found to secrete glycogen that supports an indigenous population of lactobacilli, which ferment the glycogen into lactic acid, thereby protecting the vagina from pathogenic bacteria, which cannot survive in an acid environment. “The presence of lactobacilli as a part of the normal vaginal flora is an important component of reproductive health.”19 Our indigenous bacteria protect us everywhere and enable us to function in myriad ways that are just beginning to be understood. From an evolutionary perspective, this extensive microbiota “endows us with functional features that we have not had to evolve ourselves.”20 This is a miracle of coevolution—the bacteria that coexist with us in our bodies enable us to exist. Microbiologist Michael Wilson notes that “each exposed surface of a human being is colonized by microbes exquisitely adapted to that particular environment.”21 Yet the dynamics of these microbial populations, and how they interact with our bodies, are still largely
unknown. A 2008 comparative genomics analysis of lactic acid bacteria acknowledges that research is “just now beginning to scratch the surface of the complex relationship between humans and their microbiota.”22
Bacteria are such effective coevolutionary partners because they are highly adaptable and mutable. “Bacteria continually monitor their external and internal environments and compute functional outputs based on information provided by their sensory apparatus,” explains bacterial geneticist James Shapiro, who reports “multiple widespread bacterial systems for mobilizing and engineering DNA molecules.”23 In contrast with our eukaryotic cells, with fixed genetic material, prokaryotic bacteria have free-floating genes, which they frequently exchange. For this reason, some microbiologists consider it inappropriate to view bacteria as distinct species. “There are no species in prokaryotes,” state Sorin Sonea and Léo G. Mathieu.24 “Bacteria are much more of a continuum,” explains Lynn Margulis. “They just pick up genes, they throw away genes, and they are very flexible about that.”25 Mathieu and Sonea describe a bacterial “genetic free market,” in which “each bacterium can be compared to a two-way broadcasting station, using genes as information molecules.” Genes “are carried by a bacterium only when needed . . . as a human may carry sophisticated tools.”26
The emerging details of gene transfer are fascinating. In addition to exchanging genes directly with other bacteria, bacteria have receptors to receive genes from prophages, which Sonea and Mathieu call “a unique type of biological but inanimate construction: a micro-robot for gene exchanges . . . organized like an ultra-microscopic syringe with a hollow container (‘head’), and an ultra-microscopic needle (‘tail’). . . . This exclusively bacterial type of instrument for gene exchange among living beings may be carried across large distances by water, wind, animals, etc.”
With so many mechanisms for genetic exchange, “all the world’s bacteria essentially have access to a single gene pool and hence to the adaptive mechanisms of the entire bacteria kingdom,” summarize Margulis and Sagan.27 Beyond genetic flexibility, “bacteria utilise sophisticated mechanisms for intercellular communication and even have the ability to commandeer the basic cell biology of ‘higher’ plants and animals to meet their own needs,” writes geneticist James Shapiro.28 A new understanding of bacteria is emerging; far from being simplistic “lower forms” of life, they are becoming recognized as highly evolved, with elaborate systems for adaptability and resilience.
In any particular environment, some subset of the total bacterial gene pool is present. Interesting research recently identified a new class of enzymes, produced by a marine bacteria, Zobellia galactanivorans, that can digest a polysaccharide called porphyran, found in some seaweed (including nori), where the bacteria were found. Through genome analysis, investigators identified
specific genes in the bacteria that produce the enzyme. Then, searching gene-sequencing databases, the investigators found the same genes in bacteria in the intestines of Japanese populations, but not North Americans. “This indicates that seaweeds with associated marine bacteria may have been the route by which these novel [enzymes] were acquired in human gut bacteria,” conclude the researchers, elaborating that “contact with non-sterile food may be a general factor in [enzyme] diversity in human gut microbes.”29 What this means is that, to some degree, the microbes on the food we eat determine our metabolic capabilities.
This finding raises huge questions about both the past and the future. “It remains to be determined how, during human evolution, changes in food production and preparation such as agriculture and cooking have influenced the intestinal microbiota,” notes a discussion in the journal Nature. “Consumption of hyper-hygienic, mass-produced, highly processed and calorie-dense foods is testing how rapidly the microbiota of individuals in industrialized countries can adapt while being deprived of the environmental reservoirs of microbial genes that allow adaptation by lateral transfer.”30
We need not continue to deprive ourselves!
If sterilized, processed food is starving our microbiota of genetic stimulation, live-culture foods that are rich depositories of bacterial genes are part of our human cultural legacy, everywhere. Through dietary change, we can indulge ourselves, and eat a variety of bacteria-rich living foods, precisely in order to build such genetic reservoirs inside our intestines, to enhance our metabolic capabilities, our immune function, and many other regulatory physiological functions.
Humans are not unique in having coevolved with bacterial symbionts. Plants also coevolved with and are dependent upon bacterial partners. A symbiotic relationship between photosynthesizing bacteria and other prokaryotes is thought by many to be the origin of the photosynthesizing chloroplasts in plant cells.31 The soil around plant roots makes up what is known as the rhizosphere, where plants find sustenance through elaborate interaction with a multifaceted “soil food web.”
Because we have evolved eating both plants and animals—and have coevolved with them—our coevolutionary histories encompass not only the plants and animals themselves but also their microbial associates. It is the ubiquitous presence of these life-forms, present from the very beginning but invisible until the past few centuries, which results in the ferments, nearly all prehistoric, that we love to eat and drink. The ferments, in spontaneously occurring forms, predate our consciousness of how to manipulate conditions so as to guide their development. But our consciousness did develop, and as part of that, so did the fermentation arts. The ferment itself, and our ability to produce it, is as much a product of coevolution as the person, plant, yeast, or bacterium. Thus coevolution encompasses even culture.
Notes:
3. Lynn Margulis, “Power to the Protoctists,” in Margulis and Sagan (2007), 30–31.
4. Lynn Margulis, “Serial Endosymbiotic Theory (SET) and Composite Individuality: Transition from Bacterial to Eukaryotic Genomes,” Microbiology Today 31:172 (2004); E. G. Nisbet and N. H. Sleep, “The Habitat and Nature of Early Life,” Nature 409:1089 (2001).
5. Margulis and Sagan (1986), 131–132.
6. Sorin Sonea and Léo G. Mathieu, “Evolution of the Genomic Systems of Prokaryotes and Its Momentous Consequences,” International Microbiology 4:67–71 (2001).
7. Jian Xu and Jeffrey I. Gordon, “Honor Thy Symbionts,” Proceedings of the National Academy of Sciences 100(18):10452 (2003).
8. Fredrik Bäckhed et al., “Host-Bacterial Mutualism in the Human Intestine,” Science 307:1915 (2005).
9. D. C. Savage, “Microbial Ecology of the Gastrointestinal Tract,” Annual Review of Microbiology 31:107–133 (1977).
10. Ruth E. Ley, Daniel A. Peterson, and Jeffrey I. Gordon, “Ecological and Evolutionary Forces Shaping Microbial Diversity in the Human Intestine,” Cell 124:837 (2006).
11. Steven R. Gill et al., “Metagenomic Analysis of the Human Distal Gut Microbiome,” Science 312:1357 (2006).
12. Bäckhed et al. (2005)
13. M. J. Hill, “Intestinal Flora and Endogenous Vitamin Synthesis,” European Journal of Cancer Prevention 6(Suppl. 1):S43 (1997).
14. S. C. Leahy et al., “Getting Better with Bifidobacteria,” Journal of Applied Microbiology 98:1303 (2005).
15. Lora V. Hooper et al., “Molecular Analysis of Commensal Host–Microbial Relationships in the Intestine,” Science 291:881 (2001).
16. Denise Kelly et al., “Commensal Gut Bacteria: Mechanisms of Immune Modulation,” Trends in Immunology 26:326 (2005).
17. Elizabeth Grice et al., “Topographical and Temporal Diversity of the Human Skin Microbiome,” Science 324:1190 (2009).
18. Jørn A. Aas et al., “Defining the Normal Bacterial Flora of the Oral Cavity,” Journal of Clinical Microbiology 43:5721 (2005).
19. E. R. Boskey et al., “Origins of Vaginal Acidity: High D/L Lactate Ratio Is Consistent with Bacteria Being the Primary Source,” Human Reproduction 16(9):1809 (2001).
20. Bäckhed et al. (2005)
21. Wilson (2005), 375. 22. Joel Schroeter and Todd Klaenhammer, “Genomics of Lactic Acid Bacteria,” FEMS Microbiology Letters 292(1):1 (2008).
23. J. A. Shapiro, “Bacteria Are Small But Not Stupid: Cognition, Natural Genetic Engineering, and Socio-Bacteriology,” Studies in the History and Philosophy of Biological and Biomedical Sciences 38:807 (2007).
24. Sorin Sonea and Léo G. Mathieu, “Evolution of the Genomic Systems of Prokaryotes and Its Momentous Consequences,” International Microbiology 4:67 (2001).
25. “Interview with Lynn Margulis,” Astrobiology Magazine (October 9, 2006), online at http://astrobio.net/news/modules.php?op=modload&name=News& le=article&sid=2108, accessed December 5, 2009.
26. Léo G. Mathieu and Sorin Sonea, “A Powerful Bacterial World,” Endeavour 19(3):112 (1995).
27. Margulis and Sagan (1986), 16.
28. Shapiro, 807.
29. Jan-Hendrik Hehemann, “Transfer of Carbohydrate-Active Enzymes from Marine Bacteria to Japanese
Gut Microbiota,” Nature 464:908 (2010).
30. Justin L. Sonnenburg, “Genetic Pot Luck,” Nature 464:837 (2010).
31. Margulis and Sagan (1986), 133–136.
32. Dr. Ingham made this remark September 14, 2009, at a “Soil Foodweb” workshop the author attended.
Recommended Reads
Recent Articles
Nothing says “spring” like a fresh, foraged meal! Savor the flavors of the season with this Milkweed Bud Pizza recipe.
Read MoreWhat’s so great about oyster mushrooms? First, you can add them to the list of foods that can be grown indoors! They are tasty, easy to grow, multiply fast, and they love a variety of substrates, making oyster mushrooms the premium choice. The following is an excerpt from Fresh Food from Small Spaces by R. J.…
Read MoreWant to start your own medicinal herb garden? Passionflower, lemon balm, and goldenseal are great places to begin! These herbs are jam-packed with medicinal properties and easy to grow in a majority of climates.
Read More